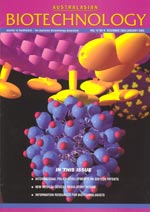
|
Australasian Biotechnology (backfiles)
AusBiotech
ISSN: 1036-7128
Vol. 8, Num. 2, 1998
|
Australasian Biotechnology,
Volume 8 Number 2, March/April 1998, pp. 91-95
Apical Membrane Antigen 1: A Leading Malaria
Vaccine Candidate
Robin F. Anders, Pauline E. Crewther and Anthony N. Hodder,
The Walter and Eliza Hall Institute of Medical Research, P.O. Royal Melbourne Hospital,
Victoria, 3050
Code Number:AU98017
Sizes of Files:
Text: 23K
Graphics: No associated graphics files
The development of a malaria vaccine remains one of the world's major public
health
priorities. Numerous proteins in several different life cycle stages have been identified as
potential vaccine components. In the asexual blood stages of Plasmodium
falciparum two
antigens, merozoite surface protein 1 (MSP1) and apical membrane antigen 1 (AMA1) stand
out as the leading vaccine candidates because of the extensive assessment of vaccines
containing these antigens in animal models of the human disease. Studies in monkeys and
mice have shown that AMA1 can induce antibody-mediated protective immune responses.
Diversity in AMA1 exists but whether this limits the efficacy of an AMA1 vaccine will need to
be examined in clinical trials carried out in areas where malaria is endemic.
Introduction
Malaria is one of the world's major public health problems with approximately 40% of the
world's population at some risk of infection (WHO, 1997). Infection with Plasmodium
falciparum causes severe morbidity in individuals lacking immunity and without
effective
treatment there is a risk of death, usually from severe anaemia or cerebral malaria. The
treatment of malaria has become more difficult because of the emergence of drug resisitant
parasites and the control of transmission has been made more difficult by the emergence of
insecticide resistant mosquito vectors. Because of this situation new tools are urgently
required to combat malaria and, therefore, the development of a vaccine against malaria is a
major priority of the World Health Organisation and other organisations addressing the health
needs of developing countries (WHO, 1996).
The complicated life cycle of the malaria parasite provides numerous points at which
induced
immune responses could prevent or limit the development of malaria parasites in their human
host. Consequently, groups involved in malaria vaccine development are focusing on antigens
expressed in sporozoites, exoerythrocytic (liver) stages, asexual blood stages, gametes and
zygotes (Engers and Godal, 1997). Numerous potential vaccine candidates have been
identified in one or other of these life-cycle stages but because there is a large number of
vaccine candidates it is difficult to find the resources to test them all in clinical trials where the
subjects are exposed to natural challenge. The development of a malaria vaccine is further
complicated by the numerous alternative strategies that now might be adopted to make a
vaccine. These different approaches: recombinant proteins, synthetic peptides, live
recombinant virus or bacteria, and naked DNA, are all being investigated by groups involved
in malaria vaccine development (Engers and Godal, 1997).
A Vaccine Against the Asexual Blood-Stages of P.
falciparum
The symptoms of malaria are caused by the synchronous development of the asexual
blood
stages of the parasite within host erythrocytes. Individuals who have developed immunity to
malaria as a consequence of naturally-acquired infections have circulating antibodies which
limit the development of the disease-causing asexual blood stages (Cohen et al.
1961)
Although studies in animal models suggest that both T- and B-cell responses are necessary for
the elimination of parasites, the reduction of parasitaemias by antibodies is likely to reduce
morbitity and mortality associated with P. falciparum infections. For these
reasons we have
been attempting to develop a recombinant protein vaccine which induces an antibody response
that limits development of the asexual blood stages of P. falciparum.
The asexual blood stages of malaria parasites are antigenically complex and it has been
assumed in the past that only a small number of the protein antigens that characterize these life
cycle stages are capable of inducing protective immune responses. The identification of
numerous antigens, either on the merozoite surface or within merozoite secretory organelles,
the mutation pattern in some of these antigens, and the evidence from a variety of model
systems indicate that the number of antigens capable of inducing protective immune responses
is larger than once thought.
Two asexual blood stage antigens, merozoite surface protein 1 (MSP1) and apical
membrane
antigen 1 (AMA1), stand out as leading vaccine candidates because they have been extensively
studied in animal models of the human infection. The identification of the genes for MSP1 and
AMA1 in many simian and rodent parasites has allowed vaccine trials to be carried out in
monkeys and mice using the antigen from simian and rodent parasites, respectively. MSP1 is a
large (~200kDa) GPI-anchored membrane protein which undergoes post-translational
processing into a number of fragments (Holder and Blackman, 1994). Because it has been
difficult to express full-length MSP1 as a recombinant protein, preclinical assessment of the
vaccine efficacy of MSP1 has focussed on fragments, particularly MSP119, a naturally
occurring C-terminal fragment which contains two "EGF-like" domains (Anders, 1997).
AMA1 is a type 1 integral membrane protein of approximately 80 kDa but like MSP1 it is
post-translationally processed to smaller fragments. The sites of cleavage in AMA1 have not
been mapped accurately however, and for the preclinical assessment of this antigen in rodents
and monkeys recombinant proteins corresponding to either the full-length protein or the
presumed ectodomain of the protein have been used. Recombinant AMA1 is currently being
prepared for a Phase I clinical trial in Brisbane and here we will briefly review the preclinical
data on which the vaccine candidacy of AMA1 is based.
Testing an AMA1 Vaccine in Animal Models
AMA1 was first identified on the surface of P. knowlesi merozoites with a
monoclonal
antibody that inhibited the invasion of rhesus erythrocytes (Deans et al.. 1982;
Deans et al.
1984). The parasite antigen (~ 66kDa), isolated by affinity chromatography from infected
rhesus erythrocytes, induced partial protection when used to immunize rhesus monkeys
(Deans et al. 1988). The results of these early studies in the P.
knowlesi/monkey model, which
first identified AMA1 as a potential vaccine component, are now supported by results from
vaccine trials using two other animal models: P. fragile in the
Saimiri monkey and P.
chabaudi in the mouse (Table 1).
Table 1: Evidence That AMA1 Can Induce
Protective Immune Response
System |
Observation |
References |
|
P. knowlesi
in
rhesus monkeys |
Mab+ to P. knowlesi AMA1 blocked
the invasion of rhesus erythrocytes by
P. knowlesi merozoites |
Deans et al. 1982 |
|
|
Rhesus monkeys partially protected
by immunization with P. knowlesi
AMA1 isolated from infected RBCs |
Deans et al. 1988
|
|
P. fragile in
Saimiri monkeys |
Recombinant P. fragile AMA1 partially
protected immunized monkeys |
Colline et al. 1994 |
|
P. chabaudi adami
in mice |
Recombinant P. chabaudi AMA1
expressed in E. coli and refolded in vitro
protected immunized mice |
Crewther et al. 1996
Anders et al. 1998 |
|
|
Parasitaemias suppressed by the passive
transfer into mice of rabbit antibodies to
P. chabaudi AMA1 |
Crewther et al. 1996
Anders et al. 1998 |
|
P. falciparum in
vitro |
Mab inhibited merozoite invasion of
human
erythrocytes |
Thomas et al.
unpublished |
|
|
Rabbit polyclonal antibodies to AMA1
inhibited merozoite invasion of human
erythrocytes |
Hodder et al.
unpublished |
+Mab; monoclonal
antibody |
In a Saimiri monkey trial in which the animals were immunized with full length
recombinant P.
fragile AMA1 produced using the baculovirus (BV) expression system, four of the five
immunized monkeys recovered without treatment whereas all control monkeys required
treatment (Collins et al. 1994) Consistent with the earlier studies in P.
knowlesi, which
indicated that protection may be mediated by anti-AMA1 antibodies, the outcome in the
immunized group of Saimiri monkeys appeared to correlate with the titre
of AMA1 antibodies
induced by immunization. All immunized monkeys developed patent parasitaemias and,
although the parasitaemias were controlled without chemotherapy, parasitaemias persisted at a
low level. The AMA1 genes for these breakthrough parasitaemias were sequenced and found
to be identical to that of the challenge inoculum.
Further preclinical assessment of AMA1 has been
carried out in the P. chabaudi/mouse model.
This more convenient model has allowed more trials to be carried out with larger numbers of
animals in the vaccine and placebo groups. Recombinant P. chabaudi AMA1
produced using
baculovirus expression protected immunized mice in one of two experiments, but more
consistent protection was obtained in mice by immunizing with the ectodomain of AMA1
expressed in E. coli and refolded in vitro (Anders et
al. 1998). The AMA1 ectodomain was
expressed with an N-terminal hexa-his tag which allowed chelate chromatography on Ni-NTA
resin to be used as a first step in the purification procedure. The P. chabaudi
AMA1
ectodomain formed inclusion bodies in E. coli and these were solubilized in 6M
guanidine-HCl
without any reducing agent prior to the Ni-chelate chromatography. The protein eluted at pH
4.5 from the Ni-NTA resin was refolded by dilution to ~50 mg/ml in a buffer containing
oxidized and reduced glutathione to generate intramolecular disulphide bonds which stabilise
the native protein. Following refolding, the protein was further purified by anion exchange
chromatography.
In three trials, two using inbred mice and one using
outbred mice, immunization with the
refolded ectodomain of P. c. adami DS strain almost abolished mortality (Table
2) and
reduced average peak parasitaemias from ~50% to 1-3% (Anders et al. 1998).
Although some
immunized mice in each experiment did not develop patent parasitaemias most developed
parasitaemias which resolved without the severe morbidity usually seen when mice are infected
with the DS strain of P. c. adami. Immunization with the refolded AMA1, which
was usually
formulated in the SEPPIC adjuvant ISA720, induced very high antibody titres to the
vaccinating antigen and, as seen in the earlier P. fragile/Saimiri
studies (Collins et al. 1994),
the titre of this response correlated with the degree of protection.
In all the AMA1 sequences that have been
determined to date there are 16 conserved cysteine
residues which form eight intramolecular disulphide bonds (Hodder et al. 1996).
The results of
extensive analyses indicated that the majority, if not all, of these disulphide bonds were in
place in the refolded P. chabaudi AMA1 used in these mouse vaccine trials
(Hodder et al.
unpublished results). The protection induced by immunizing with the refolded AMA1 was
dependent on the disulphide-bonded structure as there was no reduction in peak parasitaemias
in mice immunized with reduced and alkylated antigen(Anders et al. 1998).
When the data
from all of the trials of AMA1 in mice are combined, immunization with the reduced and
alkylated AMA1 does appear to have been associated with reduced mortality (Table 2). It may
be that T-cell priming by the reduced and alkylated antigen enhances protective AMA1
antibody responses induced by the developing parasitaemia following challenge
(Amante et al.
1997).
Table 2: Effect of Immunization with
AMA1 on Mortality in P. chabaudi-Infected
Mice+
Group |
Number |
Survivors |
%
Mortality |
Control mice |
36 |
10 |
72 |
Red. & alk. AMAl* |
17 |
11 |
35 |
Refolded AMA1 |
37 |
35 |
5 |
+See Anders et al. 1998 for details of
these trials
*The reduction and alkylation of AMA1
irreversibly destroys the intramolecular disulphide
bonds which are important for stabilizing the native conformation of AMAl.
Although AMA1 lacks the major polymorphisms
that characterize a number of the other
merozoite surface antigens, numerous point mutations have occurred in the AMA1 genes of
various Plasmodium species. In P. falciparum the majority of these
mutations are
non-synonymous, resulting in amino acid substitutions at more than 50 positions in the
sequence (Marshall et al. 1996). The bias towards non-synonymous mutations,
clustering of
the mutations, and the radical nature of the majority of the amino acid substitutions indicates
that selection, possibly by protective antibodies, has played a role in the emergence of this
sequence diversity (Crewther et al. 1996).
One reason P. chabaudi was chosen
as the parasite for use in the vaccine trials in mice was the
availability of two subspecies and several different parasite isolates. This allows testing of an
AMA1 vaccine for efficacy against challenge with heterologous as well as homologous
parasites. When mice immunized with recombinant AMA1 from the DS strain were challenged
with the 556KA strain of P. c. adami there was no protection (Crewther
et al. 1996). The DS
and 556KA AMA1 sequences differ at 36 positions which is slightly more different than the
two most different P. falciparum sequences (Marshall et al. 1994).
Antibodies raised in rabbits
to the DS AMA1 cross-reacted with the 556KA antigen but on passive transfer into mice,
these antibodies had no effect on a 556KA parasitaemia whereas they caused a marked
reduction in DS parasitaemias.
Preparing For a Human Trial With P.
falciparum AMA1
These preclinical studies in monkeys and mice have
provided the justification for testing a
recombinant AMA1 vaccine in human volunteers, but the studies in mice with heterologous
challenge indicate that diversity may limit the efficacy of an
AMA1 vaccine. We have been encouraged to find that antibodies raised in rabbits to refolded
recombinant AMA1 from the 3D7 line of P. falciparum are inhibitory in
vitro for all three
lines of P. falciparum so far examined (Hodder et al. unpublished
results). The AMA1 of one
of these lines, HB3, has 24 amino acid differences from the 3D7 sequence. Ultimately, the
efficacy of AMA1 and the other leading vaccine candidates must be tested in field trials where
the subjects will be challenged with an enormous diversity of parasite genotypes. In an
endemic setting, the majority of these parasites cause no disease and this will provide an
opportunity for the immune responses primed by immunization to be boosted by natural
infections. It is possible that such boosting will increase the titre and broaden the specificity of
the protective antibody response so that there is efficacy against infections due to parasites of
diverse genotypes.
Acknowledgements
The research on AMA1 at The Walter & Eliza
Hall Institute of Medical Research has been
funded by the National Health and Medical Research Council (Australia), the Cooperative
Research Centre for Vaccine Technology and the United Nations Development
Programme/World Bank/World Health Organisation Special Programme for Research and
Training in Tropical Diseases.
References
Amante, F.H., Crewther, P.E., Anders, R.F. &
Good, M.F. (1997) A cryptic T cell epitope on
the apical membrane antigen 1 of Plasmodium chabaudi adami can prime for an
anamnestic
antibody response. Implications for vaccine design. J. Immunol.,
159, 5535.
Holder, A.A. & Blackman, M.J. (1994) What
is the function of MSP-1 on the malaria
merozoite? Parasitol Today, 10, 182
Anders RF (1997) Vaccines against asexual blood
stages of Plasmodium falciparum. In "New
Generation Vaccines". Eds Levine MM, et al. Marcel Dekker, Inc. p. 1035.
Anders R.F., Crewther PE, Edwards S et al.
(1998) Immunisation with recombinant AMA-1
protects mice against infection with Plasmodium chabaudi.
Vaccine, 16, 240.
Cohen, S., McGregor, I.A. & Carrington,
S.C. (1961) Gamma-globulin and acquired
immunity to human malaria. Nature, 192,
733.
Collins, W.E., Pye, D, Crewther, P.E. et al. (1994)
Protective immunity induced in squirrel
monkeys with recombinant apical membrane antigen-1 of Plasmodium fragile.
Am J Trop
Med Hyg 51, 711.
Crewther, P.E., Matthew, M.L.S.M., Flegg, R.H.
and Anders, R.F. (1996) Protective immune
responses to apical membrane antigen 1 of Plasmodium chabaudi involve
recognition of
strain-specific epitopes. Infect Immun 64, 3310.
Deans, J.A., Alderson, T., Thomas, A.W. et al.
(1982) Rat monoclonal antibodies which
inhibit the in vitro multiplication of Plasmodium knowlesi.
Clin Exp Immunol 49, 297.
Deans, J.A., Knight, A.M., Jean, W.C. et al.
(1988) Vaccination trials in rhesus monkeys with
a minor, invariant, Plasmodium knowlesi 66 kD merozoite antigen.
Parasite Immunol 10, 535.
Deans, J.A., Thomas, A,W,, Alderson, T., &
Cohen, S. (1984) Biosynthesis of a putative
protective Plasmodium knowlesi merozoite antigen. Mol Biochem
Parasitol 11, 189.
Engers, H.D. & Godal, T. (1998) Malaria
vaccine development. Parasitology Today, 14, 56.
Hodder, A.N., Crewther, P.E., Matthew,
M.L.S.M. et al. (1996) The disulphide bond
structure of Plasmodium apical membrane antigen-1. J Biol Chem
271, 29446.
Marshall, V.M., Zhang, L-X., Anders, R.F. and
Coppel, R.L. (1996) Diversity of the vaccine
candidate AMA-1 of Plasmodium falciparum. Mol Biochem
Parasitol 77, 109.
WHO (1997) World Malaria Situation in 1994.
Weekly Epidemiological Record, WHO
WHO (1996) Ad Hoc Committee on Health
Research Relating to Future Intervention
Options. Investing in Health Research and Development (TDR/Gen/96.1),
WHO
Copyright 1998 Australian Biotechnology Association Ltd.
|