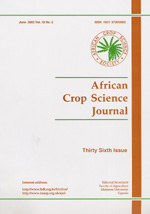
|
African Crop Science Journal
African Crop Science Society
ISSN: 1021-9730 EISSN: 2072-6589
Vol. 3, Num. 2, 1995, pp. 171-180
|
African Crop Science Journal, Vol. 3. No.2, pp. 171-180, 1995
NEW VISTAS ARE OPENED FOR SORGHUM IMPROVEMENT BY GENETIC
TRANSFORMATION
A.K. KONONOWICZ, A.M. CASAS, D.T. TOMES^1, R.A. BRESSAN and
P.M. HASEGAWA
Purdue University, 1165 Horticulture Building, West Lafayette,
IN 47907-1165, USA. 1 Pioneer Hi-Bred Intl., Inc., 7300 N.W.
62nd Avenue, P.O. Box 1004, Johnston, IA 50131-1004, USA
Code Number:CS95023
Size of Files:
Text: 41.5K
No associated graphics files
ABSTRACT
Transgenic fertile sorghum plants (Sorghum bicolor L.
Moench) were obtained by microprojectile bombardment of
immature embryo and immature inflorescence explants.
Regeneration from the calli was through embryogenesis and
organogenesis pathways, resulting in both the uniformly
transformed and chimeric plants. Plant regeneration occurred
on media supplemented with bialaphos. Microprojectile
bombardment resulted in plants resistant to the herbicide and
exhibited phosphinothricin acetyltransferase activity. The
presence of bar, uMa and luc genes in the T[o] plants
was confirmed by Southern blot analysis of genomic DNA. The
herbicide resistance was inherited in T[1] plants.
Key Words: Bialaphos, explants, herbicide,
regeneration, Sorghum bicolor, transgenic
RESUME
Les plantes transgeniques fertiles de surgho (Sorghum
bicolor L. Moench ) etaient obtenues par bombardement de
microprojectiles d'embryons immatures et d'explants
d'inflorescences immatures. La regeneration a partit de cales
a lieu a travers l'embryogenese et l'organogenese qui
resultent en la formation des plants uniformement transformes
et des chimeres. La regeneration des plantes se fait sur des
milieux ayant obtenu un supplement de bialaphos. Le
bombardement des microprojectiles a abouti a la production des
plants resistants aux herbicides et presentant l'activite
phosphinothricine acetyltransferase.
La presence des genes bar, uida et luc sur les plants T[o] a
ere confirmee a l'aide de l'analyse southern blot du DNA
genomique. La resistance a l'herbicide etait herite par les
plants en T[1].
Mots Cids: Bialaphos, explants, herbicide,
regeneration, Sorghum bicolor; transgenique
INTRODUCTION
The development of methods for genetic transformation of
plants has made genes that are derived from recombinant DNA
technologies accessible for practical use. This has allowed
the genetic improvement of particular crop species by the use
of genes obtained from totally unrelated species. The
application of these methods has produced transgenic plants
that are resistant to various bacterial (Martin et al.,
1993), viral (Cuozzo et al., 1988; Nelson et al,
1988; Lawson et al., 1990) or fungal infections
(Broglie et al., 1991; Liu et al, 1994),
resistant to insect pests (Fischhoff el al., 1987;
Vaeck et al., 1987; Perlak et al., 1993),
tolerant to the application of various herbicides (Shah et
al., 1986; De Greef et al., 1989), are male sterile
(Mariani et al., 1990; Denis et al., 1993), have
a higher nutritional content in the seed (Altenbachetal,
1989), have delayed fruit-ripening (Oeller et al.,
1991), or have a greater degree of stress protection
(Tarczynsk et al. 1993), due to the introgression of
new genes.
The transformation of cereals has been delayed for many
years by the lack of efficient transformation systems.
Agrobacterium tumefaciens, the highly effective
vector for dicotyledonous crops until recently (Hiei et al.,1974)
either did not infect monocotyledonous plants, or did so very
inefficiently (Gould et al., 1991; Shen et al., 1993),as
described for asparagus (Bytebier et al., 1987) or rice
(Reineri et al., 1990). Mediated by PEG (Lorz et al.
1985) or electropotation (Fromm et al., 1985), the
introduction of DNA into protoplasts has been extensively
examined for many cereals (Rhodes et al. 1988;
Shimamoto et al., 1989; Datta et al., 1990). However.
plant regeneration from protoplasts is very laborious, is
strongly genotype dependent and apparently is only routinely
applicable in the case of rice (Hayashimoto et al.,
1990; Peng et al.. 1992).
Because of difficulties in obtaining transgenic
monocotyledonous plants using Agrobacterium or
protoplasts, other approaches have been attempted for
introducing DNA into plant cells (Songstad et al.,
1995). These include macroinjection (De la Pena et al.
1987), embryo imbibition (Topfer et al., 1990),
agroinfection with gemini ,viruses (Grimsley et al.,
1988), the pollen tube absorption pathway (Luo and Wu,
1988; Langridge et al., 1992), silicon-carbide fiber
(Kaeppler et al., 1992), tissue electropotation
(D'Halluin et al., 1992; Xu and Li, 1994), and
microprojectile bombardment (Sanford, 1988; Klein et al.,
1992; Christou, 1993; Morrish et al. 1993; Sanford
et al., 1993). Of all these techniques particle
bombardment has proved to be the most successful and
reproducible, leading to the production of transgenic plants
of a wide range of species, including most of the cereals and
among them Sorghum bicolor (L. Moench) Casas et al.,
1995; Maheshwarietal.,l995; Vasil 1995). This methodology
allows the introduction of DNA into live cells or tissues,
making it possible to evaluate transient expression of
different gene constructs in intact organs, as well as the
recovery of stably transformed tissues and plants.
DNA delivery utilizing microprojectile bombardment
involves the acceleration of metal particles coated with DNA,
such that they penetrate the cell wall and enter the cell
proper. Bombarded cells transiently express the introduced
gene and some of these cells incorporate the gene into the
plant genome and become stably transformed. One can then
select then select a population of transformed cells and
eventually regenerate plants. One advantage of this
methodology is that it can be used to target a wide range of
tissues or organs, such as cell suspensions (Fromm et
al., 1990; Gordon-Kamm et al. 1990; Cao et al.,
1992; Somers et al., 1992) or calli (Bower and
Birch, 1992; Vasil et al., 1992), immature embryos
(Christou et al.. 1991; Casas et al., 1993; Weeks et
al., 1993; Becker et al., 1994; Nehra et al., 1994;
Wan and Lemaux, 1994). meristems (Bilang et al., 1993;
Perez-Vicente et al.,1993; Ritala et al., 1994),
pollen (Twell et al., 1989; Hamilton et al.,
1992), microspore derived embryos (Creissen et al., 1990;
Wan and Lemaux, 1994), tassel primordia (Dupuis and Pace,
1993), or leaf tissue (Reggiardo et al., 1991;
Gallo-Meagher and Irvine. 1993). Using those various target
tissues, stable transformation of all the major cereals
including sorghum (Casas et al., 1995) has been
accomplished.
Microprojectile mediated transformation systems consist of
four major components. All of them are apparently essential
for successful transformation. These include: l)a tissue
culture component, 2) DNA delivery, 3).selection and 4)
molecular and genetic analyses of recovered plants.
This paper focuses on a transformation system that was
developed in our laboratory and resulted in stable
transformation of Sorghum bicolor utilizing
microprojectile bombardment and selection under bialaphos
selection pressure.
TISSUE CULTURE COMPONENT
The choice of the explant or target tissue for
transformation and subsequent regeneration are the most
critical factors influencing transformation via
microprojectile bombardment. Over the last 20 years, several
relatively efficient plant regeneration protocols have been
developed for sorghum. These include regeneration from both
immature and mature zygotic embryos (Gamborg et al..
1977; Dunstan et al., 1978, 1989; Brar et al.,
1979; Mackinnon et al.. 1986; Cai et al.,
1987; Ma et al., 1987), from shoot segments of
germinating seeds (Brar et al., 1979), from immature
inflorescences (Bretell et al., 1980; Boyes and Vasil,
1984; Cai and Butler? 1990), and shoot tips (Bhaskaran and
Smith, 1988; 1989; Bhaskaran et al., 1988). In our
transformation research. immature embryos and immature
inflorescences were utilized as explants for particle
bombardment and plant regeneration. Protocols for plant
regeneration via the embryogenesis pathway from both types of
explants have been developed in Dr. L.G. Butler's (Dept. of
Biochemistry, Purdue University, West Lafayette, Indiana, USA)
laboratory, and after necessary modifications used in our
laboratories to produce transgenic sorghum plants (Casas et
al., 1993; 1995).
The plant regeneration protocol from immature embryos
includes culture of immature zygotic embryos (12-15 days after
pollination) onto a basal medium containing Murashige and
Skoog (Murashige and Skoog, 1962) salts, modified B5 vitamins
(Gamborg et al., 1977) and agar (8 g 1^-1) supplemented
with asparagine (150mg l^-1), 10% coconut water, 2,4-D (2 mg
l^-1), and sucrose (30 g 1^-1) ( 16 medium) for induction of
embryo genesis and initiation of embryogenic callus. Selection
and maintenance of embryogenic tissue and shoot and root
development from organized structures are as described in
details by Cai and Butler (1990). Media contains the basal
constituents supplemented with 2 mg l^-1 2,4-D, 0.5 g l^-1
kinetin, 30 g 1^-1 sucrose for maintenance of embryogenic
tissue ($2 medium) or 1 mg l^-1 IAA, 0.5 mg 1
kinetin, and 20 g l^-1 sucrose to facilitate shoot
development. For root development, plantlets are transferred
to RM medium containing 0.5 X Murashige and Skoog salts, 0.5
mg l^-1 NAA, 0.5 mg 1^-1 IBA, 20 g l^-1 sucrose and 8 g 1^-1
agar. Cultures of immature embryos and embryogenic tissue are
grown in darkness, at 26 C and recultured every 2 weeks.
Tissues on shoot regeneration medium are subcultured every 4
weeks and grown at 26^-1 C under a 16-hr photoperiod
(1000-2000 lx from fluorescent, cool white light).
The plant regeneration protocol from immature
inflorescences includes isolation of immature inflorescences
(0.5 to 3 cm long) and after cutting into 0.5 cm large pieces,
culture onto NS2 medium consisting of Murashige and Skoog (MS)
salts (Murashige and Skoog, 1962), B5 vitamins (Gamborg et
al., 1977) 2.5 mg 1^-10 2,4-D, 0.5 mg l^-1 kinetin, 60 g
1^-1 sucrose. and 7 g l^-1 agar to induce embryogenic callus.
Embryogenic callus can be maintained and plants from
embryogenic tissue regenerated under culture conditions as
described for immature embryo explants. After acclimation to
low humidity, regenerated plants are transferred to a
greenhouse and there grown to maturity.
It has to be emphasized. however, that genotype specific
differences in responsiveness of primary explants to
regeneration protocols have been found among sorghum genotypes
utilized in our studies. The best responsive genotypes have
been selected for further transformation research that
utilized both types of explants, e.g. immature embryos
(PS98012) and immature inflorescences (SRN39).
DNA DELIVERY BY PARTICLE BOMBARDMENT
Besides the importance of target explant and plant
regeneration, there are several features of the particle gun
that influence the efficiency of the transformation process.
These include the type, size and amount of microprojectiles
used, the velocity of the microprojectiles and target
distance, the amount of DNA per shot and the number of
bombardments. Transient expression of reporter genes can be
used to optimize these bombardment parameters. The pressure
and expression of commonly used reporter genes can be
determined by different methods. For the uida gene
encoding for B-glucuronidase, histochemical or fluorometric
assays described by Jefferson et al. ( 1986, 1987) are
used. Furthermore, radiochemical or chemiluminescence methods
are used to detect chloramphenicol acetyltransferase (Fromm
et al. 1985) or luciferase reporter genes (Ow et
al., 1986; Olssonet al., 1989), respectively.
Another group of reporter genes includes transcriptional
activators that regulate anthocyanin metabolism in maize, e.g.
R (Ludwig et al., 1989), Cl Paz-Ares et al.
1987) and B-Peru (Chandler et al., 1988).
The production and accumulation of anthocyanin pigment in a
given tissue depends on the presence of a functional set of
regulatory genes, which include Al, Bzl and C2.
Expression of these genes can be easily monitored visually
and, most important, nondestructively (Ludwig et al.,
1990; Bowen, 1992).
TABLE 1. Particle bombardment parameters
Immature embryos:
Size of explants: 1-2 mm immature embryos
DNA amount: 0.15 ug of each plasmid DNA for cotransformation
0.3 ug of DNA when single plasmid is used
Tungsten particles:
size: 1.7 um (M25)
amount: 0.15 mg
Pressure: 1100 PSI
Distance: 4.5 cm for single shot
7.5 cm for double bombardment
Immature inflorescences:
Size of H: 1-3 cm
DNA amount: 0.2 ug of each plasmid DNA for co-
transformation
0.4 4g of DNA when single plasmid is used
Tungsten particles:
size: 1.7 um (M25)
amount: 0.15 mg
Pressure: 1300 PSi
Distance: 4.5 cm for single shot
7.5 cm for double bombardment
TABLE 2. Analysis of Transformation Event's T[o] Plants
Event Number of insertions Phenotypic
expression
--------------------- -----------
bar luc bar luc
-------------------------------------------------------------
1119 (fertile) l(EcoR l/bar) NA yes NA
1409 (sterile) 2 (EcoR ll/bar) NA yes NA
1702-2H (fertile) (EcoR ll/bar)
(Sac ll/bar) NA yes NA
1752 (male sterile) 2 (EcoR l/bar) NA yes NA
2251 (fertile) 2 (Sac ll/bar) 1 (EcoRI/luc) yes no
2441 (fertile) 3 (Sac ll/bar) 3 (EcoRI/luc) yes yes
NA - not applicable since bombardment plasmids did not contain
this gene
Both, uidA gene and R/Cl transcriptional
regulators (driven by single or double CaMV 35S promoter with
maize Adhl gene intron) have been successfully used in
our sorghum transformation research as reporter genes to
optimize particle bombardment parameters (Table 1) (for review
see Sanford et al., 1993) and later for screening
bombarded explants during early stages of plant
regeneration.
SELECTION STRATEGY
A reliable selection procedure is a prerequisite for
development of an efficient transformation system. An
important feature of the selection system is its ability to
facilitate the emergence of a developing transformant enclosed
in a mass of untransformed tissue. As mentioned previously,
both immature embryos and immature inflorescences are used as
primary explants and plants are regenerated through in
vitro induced somatic embryogenesis. For each type of
explant, it is critical to develop an efficient selection
strategy that utilizes selectable markers and an appropriate
selective agent, antibiotic or herbicide, to identify and
multiply transformed cells. Considering the probability that
only a small percentage of the transiently expressing cells
are likely to be stably transformed, it is important to use
selection that confers a growth advantage to the transformed
cells. To impose an efficient selection pressure, while
maintaining the competence for regeneration, the dose, mode
and time of selective agent application, as well as the
duration of the selection need to be considered.
Several selectable marker genes conferring resistance to
either antibiotics or herbicides have been isolated and are
currently used for plant transformation (Table 2)(for review
see Wilmink and Dons, 1993; Hinchee et al., 1994). In
the case of monocotyledonous plants, the most common selection
systems involves the use of the bacterial genes nptll, hph
and bar that confer resistance to kanamycin,
hygromycin and phosphinothricin, respectively. Even though
kanamycin has been used to recover transformed calli and
plants (Murry et al., 1993), cells of many
monocotyledonous plants appeared to be quite resistant to this
antibiotic (Klein et al., 1989; Hagio et al.,
1991; Vasil et al., 1991). Better results have been
obtained using geneticin (Bower and Birch, 1992; Nehra et
al., 1994) and hygromycin (Christou et al., 1991;
Walters et al., 1992). Nevertheless, the herbicides
bialaphos or Basta were proven to be the best selective agents
for cereal transformation and for this reason they were used
in our early studies on sorghum and a selection protocol
utilizing bar gene has been established in our
laboratories for regeneration of transgenic plants from
immature embryos and immature inflorescences.
As mentioned earlier the selection strategy is a
compromise that involves the level of explant organization,
the concentration of selective agent used, and the duration of
selection pressure application. A selection strategy for each
sorghum genotype has been developed experimentally based on
detailed analyses of susceptibility of non-bombarded and
bombarded explants to different doses of bialaphos at
different stages of the regeneration process. For immature
embryos we found that bialaphos at a concentration of 3 mg l^-
1 while applied at the beginning of the selection process
would severely inhibit callus formation in bombarded material,
probably due to accumulation of ammonia in the surrounding
non-transformed cells. It was necessary to initiate selection
on medium with 1 mg l^-1 of this herbicide. The formation of
embryogenic callus from immature embryos on induction medium
in the presence of a low concentration of bialaphos could be
used as an indicator of possible transformants, since
non-responsive embryos do not produce any callus later. A
minimum of three more transfers on medium with increased
concentration of bialaphos (3 mg l^-1), 2 weeks each, appeared
to be necessary to identify putative transgenic callus. It
became clear that maintenance of a continuous selection
pressure is a critical factor to recover transgenic plants,
because the sensitivity to the selective agent changes during
cell growth and differentiation. It was frequently reported
also for other plant species (Fromm et al., 1990;
Christou et al., 1991; Bower and Birch, 1992) that
plant regeneration in the absence of selection could result in
the preferential recovery of escapes, untransformed material
in large numbers. Furthermore, it has been proven that the
process of root formation and elongation is one of the most
sensitive stages of growth, and the application of selection
pressure during this stage of culture allows the
discrimination of true transgenic material (Cao et al.,
1992; Somers et al., 1992; Li et al., 1993;
Weeks et al., 1993; Wan and Lemaux, 1994). For this
reason we included bialaphos also in regeneration (3 mg l^-1)
and rooting (1 mg l^-1) media. Utilizing regeneration and
bialaphos selection protocols described above, several
transgenic sorghum plants have been regenerated in our
laboratories from both immature embryo and immature
inflorescence explants and characterized by molecular
(Southern analysis) and biochemical (PAT. GUS and luciferase
activity assays) methods. Furthermore, stability of the
introduced genes in the following generations has been
also proven using the same methodology as for primary
transformants.
Although a selection system utilizing bar gene and
bialaphos has proven to be reliable. it cannot be considered
as a system for routine sorghum transformation, because of
sorghum's ability to readily outcross with weed species. For
this reason, selection strategy is one of the priorities of
our current research. The nptll and hph genes
conferring resistance to kanamycin and hygromycin,
respectively, are now being considered as alternative
selectable markers. Introduction of agronomically important
genes (traits) and selectable marker genes into the plant
genome by cotransformation of two separated plasmids, followed
by segregating out the selectable marker gene in the progeny
is considered as another strategy. Our preliminary studies on
applying these strategies for sorghum are encouraging.
ACKNOWLEDGEMENTS
Sorghum research was supported by Pioneer HiBred International
(Johnston, Iowa) and The Consortium for Plant Biotechnology
Research, IIIc.
REFERENCES
Altenbach, S.B., Pearson, K.W., Meeker, G., Staraci,L.C.
and Sun, S.S.M. 1989. Enhancement of the methionine content
of seed proteins by the expression of a chimeric gene encoding
a methionine-rich protein in transgenic plants. Plant
Molecular Biology 13:513-522.
Becker,. D., Brettschneider, R. and Lorz, H. 1994. Fertile
transgenic wheat from microprojectile bombardment of scutellar
tissue. Plant Journal 5:299-307.
Bhaskaran, S. and Smith, R.H. 1988. Enhanced somatic
embryogenesis in Sorghum bicolor from shoot tip
culture. In Vitro Cellular Development Biology
21:65-70.
Bhaskaran, S. and Smith. R.H. 1989. Control of morphogenesis
in sorghum by 2,4-Dichlorophenoxyacetic acid and cytokinins.
Annual Botany 64:217-2214.
Bhaskaran, S., Neumann, A.J. and Smith, R.H. 1988. Origin of
somatic embryos from cultured shoot tips of Sorghum bicolor
(L.) Moench. In Vitro Cellular Development Biology
24:947- 950.
Bilang, R., Zhang, S., Leduc, N., Iglesias, V.A., Gisel, A.,
Simmonds, J. Potrykus, I. and Sautter, C. 1993. Transient gene
expression in vegetative. shoot apical meristems of wheat
after ballistic microtargeting. Plant Journal
4:735-744.
Bowen, B. 1992. Anthocyanin genes as a visual markers in
transformed maize tissues. In: GUS Protocols: Using the GUS
Gene as a Reporter of Gene Expression. Gallagher. S.R.
(Ed.), pp. 163-177. Academic Press, Inc, San Diego.
Bower, R. and Birch, R.G. 1992. Transgenic sugarcane plants
via microprojectile bombardment. Plant Journal
2:409-416.
Boyes, C.J. and Vasil, I.K. 1984. Plant regeneration by
somatic embryogenesis from cultured young inflorescences of
Sorghum arundinaceum (Desv.) Stapf. var. Sudanese
(Sudan grass). Plant Science Letters 35:153-57.
Brar, D.S., Rambold, S., Gamborg, O. and Constabel, F. 1979.
Tissue culture of corn and sorghum. Z. pflanzenphysiology
95:377-88.
Brettell, R.I.S., Wernicke, W. and Thomas, E. 1980.
Embryogenesis from cultured immature inflorescences of
Sorghum bicolor. Potoplasma 104:141 - 148.
Broglie, K., Chet. I., Holliday, M., Cressman, R., Biddie, P.,
Knowlton, S., Mauvais, C.J. and Broglie, R. 1991. Transgenic
plants with enhanced resistance to the fungal pathogen
Rhizoctonia solani. Science 254:1194-1197.
Bytebier, B., DeBoeck, F., De Greve, H., Van Montagu, M. and
Hernalsteens, J.P. 1987. TDNA organization in tumor cultures
and transgenic plants of the monocotyledon Asparagus
officinalis. Proceedings of the National Academy of Sciences,
USA 84:5345-5349.
Cai, T. and Butler, L. 1990. Plant regeneration from
embryogenic callus initiated from immature inflorescences of
several high-tannin sorghums. Plant Cell, Tissue and
Organ
Culture 20:101-110.
Cai, T. Daly, B. and Butler. L. 1987. Callus induction and
plant regeneration from shoot portions of mature embryos of
high-tannin sorghums. Plant Cell, Tissue and Organ
Culture 9:245-252.
Cao, J., Duan, X., McElroy, D. and Wu, R. 1992.
Regeneration of herbicide resistant transgenic rice
plants following microprojectile-mediated transformation
of
suspension culture cells. Plant Cell Reproduction
11:586-591.
Casas, A.M., Kononowicz, A.K-, Zehr, U.B., Tomes, D.T.,
Axtell, J.D., Butler, L.G., Bressan, R.A. and Hasegawa, P.M.
1993. Transgenic sorghum plants via microprojectile
bombardment. Proceedings of National Academy of Sciences,
USA 90:11212-11216.
Casas, A.M., Kononowicz, A.K., Bressan, R.A. and Hasegawa,
P.M. 1995. Cereal transformation through particle
bombardment. Plant Breeding Review 13:231-260.
Chandler, V.L. Radioella, J.p., Robbins, T.P, Chen, J. and
Turks, D. 1988. Two regulatory genes of the maize anthocyanin
pathway are homologous: Isolation of B utilizing R genomic
sequences. Plant Cell 1:1175-1183.
Christou, p. 1993. Particle gun mediated transformation.
Current Opinion in Biotechnology 4:135-141.
Christou, P., Ford, T.L. and Kofron, M. 1991. Production of
transgenic rice (Orvza sativa L.) plants from
agronomically important indica and japonica varieties
via electric discharge particle acceleration of exogenous DNA
into immature zygotic embryos. Bio/Technology 9:957-
962.
Creissen, G., Smith, C., Francis, R., Reynolds, H. and
Mullineaux, P. 1990. Agrobacterium and microprojectile-
mediated vital DNA delivery into barley microspore-derived
cultures. Plant Cell Reporter 8:680-683.
Cuozzo, M., O'Connel, K.M., Kaniewski, W., Fang, R., Chua, N.
and Tumer, N.E. 1988. Viral protection in transgenic tobacco
plants expressing the cucumber mosaic virus coat protein or
its antisense RNA. Bio/Technology 6:549-555.
Datta, S .K., Peterhans, A. Datta, K. and Potrykus,
1990. Genetically engineered fertile indica rice recovered
from protoplasts. Bio/Technology 8:736-740.
De Greef, W., Delon, R., De Block, M., Leemans, J. and
Botterm-nan, J. 1989. Evaluation of herbicide resistance in
transgenic crops under field conditions. Bio/Technology
7:61-64.
De La Pena, A., Lorz, H. and Schell, J. 1987. Transgenic rye
plants obtained by injecting DNA into young floral tillers.
Nature 325:274-276.
Denis, M, Delourme, R., Gourmet, J.p., Mariani, C. and Renard,
M. 1993. Expression of engineered nuclear male sterility in
Brassica napus. Genetics, morphology, cytology, and
sensitivity to temperature. Plant Physiology
101:1295-1304.
D'Halluin, K., Bonne, E, Bossut, M., De Beuckeleer, M. and
Leemans, J. 1992. Transgenic maize plants by tissue
electroporation. Plant Cell 4: 1495-1505.
Dunstan, D.I., Short, K.C. and Thomas, E. 1978. The anatomy of
secondary morphogenesis in cultured scutellum tissues or
Sorghum bicolor. Protoplasma 97:251-260.
Dunstan, D.I., Short, K.C., Dhaliwal, H. and Thomas, E. 1979.
Further studies on plantlet production from cultured tissues
of Sorghum bicolor. Protoplasma 101:355-361.
Dupuis, I. and Pace, G.M. 1993. Gene transfer to maize male
reproductive structure by particle bombardment of tassel
primordia. Plant Cell Reproduction 12:607-611.
Fischhoff, D.A., Bowdish, K.S. Perlak, F.J., Marrone, P.G.,
McCormick. S.M., Niefermeyer, J.G, Dean, D.A., Kusano-
Kreetzmer, K., Mayer, E.J., Rochester, D.E., Rogers, S.G. and
Fraley, R.T. 1987. Insect tolerant transgenic tomato plants.
Bio/Technology 5:807-813.
Fromm, M.E., Taylor, L.P. and Walbot, V. 1985. Expression of
genes transferred into monocot and dicot plant cells by
electroporation. Proceedings National Academy of Sciences,
USA 82:5824-5828.
Fromm, M.E., Morrish, F., Armstrong, C., Williams, R., Thomas,
J. and Klein, T.M. 2990. Inheritance and expression of
chimeric genes in the progeny of transgenic maize plants.
Bio/Technology 8:833-839.
Gallo-Meagher, M. and Irvine, J.E. 1993. Effects of tissue
type and promoter strength on transient GUS expression in
sugarcane following particle bombardment. Plant Cell
Reproduction 12:666-670.
Gainborg, O.L., Shyluk, J.P. Brar, D.S. and Constabel,
F. 1977. Morphogenesis and plant regeneration from callus of
immature embryos of sorghum. Plant Science Letters
100:67-74.
Gordon-Kamm, W.J., Spencer, TM, Mangano, M.L., Adams, T.R.,
Daines, R.J., Start, W.G., O'Brien, J.V., Chambers,
S.A.,Adams, Jr, W.R., Willetts, NG, Rice, T.B., Mackey,
C.J., Krueger, R.W., Kausch, A.P. and Lemaux, P.G. 1990.
Transformation of maize cells and regeneration of fertile
transgenic plants. Plant Cell 2:603-618.
Gould, J., Devey, M., Hasegawa, 0., Ulian, E.C., Peterson, G.
and Smith, R.H. 1991. Transformation of Zea mays L.
using Agrobacterium tumefaciens and the shoot apex.
Plant Physiology 95:426-434.
Grimsley, N.H., Ramos, C., Hein, T. and Hohn, B. 1988.
Meristematic tissues of maize plants are most susceptible to
agroinfection with maize streak virus. Bio/Technology
6:185-188.
Hamilton, D.A., Roy, M., Rueda, J., Sindhu, R.K., Sanford, J.
and Mascarenhas, J.P. 1992. Dissection of a pollen-specific
promoter from maize by transient transformation assays.
Plant Molecular Biology 18:211-218.
Hayashimoto, A., Liu, Z. and Mural, N. 1990. A polyethylene
glycol-mediated protoplast transformation system for
production of fertile transgenic rice plants. Plant
Physiology 93:857-863.
Hiei, Y., Ohta, S., Komari, T. and Kumashiro, T. 1994.
Efficient transformation of rice (Oryza satira L.)
mediated by Agrobacterium and sequence analysis of the
boundaries of the FDNA. Plant Journal 6:271-282.
Hinchee, M.A.W., Corbin, D.R., Armstrong, Ch. L., Fry, J.E.,
Sato, S.S., DeBoer, D.L., Petersen, W.L., Armstrong, T.A.,
ConnorWard, D.V., Layton, J.G., and Horsch, R.B., 1994. Plant
Transformation. In: Plant Cell and Tissue Culture.
Vasil, I.K. and Thorpe , T.A. (Eds.), pp. 231-270. Kluwer
Academic Publishers, Dordrecht.
Jefferson, R.A., Burgess, S.M. and Hirsch, D. 1986.
B-Glucuronidase from Escherichia coil as a gene-fusion
marker. Proceedings of the National Academy Sciences,
USA 83: 8447-8451.
Jefferson, R.A., Kavanagh, T.A. and Bevan, M.W. 1987. GUS
fusions: B-glucuronidase as a sensitive and versatile gene
fusion marker in higher plants. EMBO Journal 6:3901-
3907.
Kaeppler, H.F., Somers, D.A., Rines, H.W. and Cockbum, A.F.
1992. Silicon carbide fiber-mediated stable transformation of
plant cells. Theoretical Applied Genetics 84:560-
566.
Klein, T.M., Arentzen, R., Lewis, P.A. and Fitzpatrick-
McElligott, S. 1992. Transformation of microbes, plants and
animals by particle bombardment. Bio/Technology
10:286-291.
Landgridge, P., Brettschneider, R., Lazzeri, P. andLorz, H.
1992. Transformation of cereals via Agrobacterium and
the pollen pathway: a critical assessment. Plant Journal
2:631-638.
Lawson, C., Kaniewski, W. Haley, L., Rozman, R., Newell, C.,
Sanders, P. and Tumer, N. 1990. Engineering resistance to
mixed virus iufection in a commercial potato cultivar:
resistance to potato virus X and potato virus Y in a
transgenic Russet Burbank. Bio/ Technology
8:127-134.
Liu, D, Raghothama. K.G., Hasegawa, P.M. and Bressan, R.A.
1994 Osmotin overexpression in potato delays development of
disease symptoms. Proceedings National Academy of Sciences,
USA 91:1888-1892.
Lorz, H. Baker, B. and Schell. J. 1985. Gene transfer to
cereal cells mediated by protoplast transformation.
Molecular and General Genetics 199: 178-182.
Ludwig, S.R., Habera, L.F., Dellaporta, S .L. and Wessler,
S.R. 1989. Lc, a member of the maize R gene family responsible
for tissuespecific anthocyanin production, encodes a protein
similar to transcriptional activators and contains the
myc-homology region. Proceedings of the National Academy
Sciences, USA 86:7092-7096.
Ludwig, S.R., Bowen, B., Beach, L. and Wessler, SR. 1990. A
regulatory gene as a novel visible marker for maize
transformation. Science 247:449-450.
Luo, Z. and Wu, R. 1988. A simple method for the
transformation of rice via the pollen-tube pathway. Plant
Molecular Biology and Reproduction 6: 165-174.
Ma. H., Gu, M. and Liang, G.H. 1987. Plant regeneration from
cultured immature embryos of Sorghum bicolor (L.)
Mocnch, Theoretical Applied Genetics 73:389-394.
MacKinnon, C., Gunderson, G. and Nabors, M.W. 1986. Plant
regeneration by somatic embryogenesis from callus cultures of
sweet sorghum. Plant Cell Reproduction 5:349-351.
Maheshwari, N., Raiyalakshmi, K., Baweja, K., Dhtr, S.K.,
Chowdhry, C.N. and Maheshwari, S.C. 1995. In vitro
culture of wheat and genetic transformation - retrospect
and prospect. Critical Review of Plant Sciences
14:149-178.
Mariani, C., De Beuckeleer, M., Turettner, J., Leemans, J. and
Goldberg, R.B. 1990. Induction of male sterility in plants by
a chimaeric ribonuclease gene. Nature 347:737-741.
Martin, G.B., BrommonschenkeI, S.H., Chungwongse, J., Frary,
A., Ganal, M.W., Spivey, R., Wu, T., Earle, E.D. and Tanksley,
S.D. 1993. Map-based cloning of a protein kinase gene
conferring disease resistance in tomato. Science 262:
1432-1436.
Morrish, F., Songstad, D.D., Armstrong, C.L. and Fromm, M.
1993. Microprojectile bombardment: A method for the production
of transgenic cereal crop plants and the functional analysis
of genes. In: Transgenic Plants. Fundamentals and
Applications. Andrew Hiatt (Ed.), pp. 133-171, Marcel
Dekker Inc, New York.
Murashige, T. and Skoog, F. 1962. A revised medium for rapid
growth and bioassays with tobacco tissue cultures.
Physiology of Plants 15:473-497.
Nehra, N.S., Chibbar, R.N., Leung, N., Caswell, K., Mallard,
C., Steinhauer, L., Baga, M. and Kartha, K. 1994. Self-fertile
transgenic wheat plants regnerated from isolated scutellar
tissues following microprojectile bombardment with two
distinct gene constructs. Plant Journal 5:285-297.
Nelson, R.S., McCormick, S.M., Delannay, X., Dube,P., Layton,
J., Anderson, E.J., Kaniewska, M., Proksch, R., Horsch, R.,
Rogers, S.G., Fraley, R.T. and Beachy, R.N. 198 8.
Virus tolerance, plant growth, and field performance of
transgenic tomato plants expressing coat protein from tobacco
mosaic virus. Bio/Technology 6:403-409.
Oeller, P.W., Min-Wong, L., Taylor, L.P., Pike, D.A and
Theologis, A. 1991. Reversible inhibition of tomato fruit
senescence by antisense RNA. Science 254:437-439.
Olsson, 0., Escher, A., Sandberg, G., Schell, J., Koncz, C.
and Szalay, A. 1989. Engineering of bacterial luciferase by
fusion of luxB genes in Vibrio harveyi. Gene
81:335-347.
Ow, D., Wood, K.V., DeLuca, M., De Wet, J.R., Helinski, D.R.
and Howell, S.H. 1986.Transient and stable expression of the
firefly luciferase gene in plant cells and transgenic plants.
Science 234:856-859.
Paz-Ares, J., Ghosal, D., Wienand, U., Peterson, P.A. and
Saedler, H. 1987. The regulatory CI locus of Zea
mays encodes a protein with homology to myb
proto-oncogene products and with structural
similarities to transcriptional activators. EMBO Journal
6:3553-3558.
Peng, J., Kononowicz, H. and Hodges, T.K. 1992. Transgenic
indica rice plants. Theoretical Applied Genetics
83:855-863.
Perez-Vicente, R., Wen, X.D., Wang, Z.Y., Leduc, N., Sautter,
C., Wehrli, E., Potrykus, I. and Spangenberg, G. 1993.
Culture of vegetative and floral meristems in ryegrass:
Potential targets for microballistic transformation.
Journal of Plant Physiology 142:610-617.
Peflak, F.J., Stone, T.B., Muskoff, Y.M., Petersen, L.J.,
Parker, G.B., McPherson, S.A., Wyman, J., Love, S.,
Reed, G., Biever, D. and Fischhoff, D.A. 1993. Genetically
improved potatoes: protection from damage by Colorado potato
beetles. Plant Molecular Biology 22:313-321.
Raineri, D.M. Bottino, P., Gordon, M.P. and Nester, E.W. 1990.
Agrobacterium-mediated transformation of rice (Oryza sativa
L. ). Bio/Technology 8:33-38.
Reggiardo, M.I., Arana, J.L., Orsaria, L.M., Permingeat, H.R.,
SpitteIer, M.A. and VaIlejos, R.H. 1991. Transient
transformation of maize tissue by microprojectile
bombardment. Plant Science 75:237-243.
Rhodes, C.A., Pierce, D.A., Metler, I.J., Mascarenhas, D. and
Detmer, J.J. 1988. Genetically transformed maize plants from
protoplasts. Science 240:204-207.
Ritala, A., Aspegren, K., Kurten, U., SalmenkallioMarttila,
M., Mannonen, L., Hannus, R., Kauppinen, V., Teeri, T.H. and
Enari, T.M. 1994. Fertile transgenic barley by particle
bombardment of immature embryos. Plant Molecular Biology
24:317-325.
Sanford, J.C. 1988. The biolistic process. Trends in
Biotechnology 6:299-302.
Sanford, J.C., Smith, F.D. and Russell, J.A. 1993.
Optimizing the biolistic process for different biological
applications. Methods in Enzymology 217:483-509.
Shah, D., Horsch, R.B., Klee, H.J. Kishore, G.M., Winter,
J.A.,Tumer, N.E., Hironaka, C.M., Sanders, P.R., Gasset, C.S.,
Aulent, S. Siegel, N.R. Rogers, S.G. and Fraley, R.T. 1986.
Engineering herbicide tolerance in transgenic plants.
Science 233:478-481.
Shen, W.H., Escurado, J., Schlappi, M., Ramos, C., Hohn, B.
and Koukolikova-Nicola, Z. 1993. T-DNA transfer to maize
cells: Histochemical investigation of B-Glucuronidase
activity in maize cells. Proceedings of the National
Academy Sciences, USA 90: 1488-1492.
Shimamoto, K, Terada, R., Izawa, T. and Fujimoto, H.
1989. Fertile transgenic rice plants regenerated from
transformed protoplasts. Nature 338:274-276.
Somers, D.A., Rines, H.W., Gu, W., Kaeppler, H.F. and
Buschnell, W.R. 1992. Fertile, transgenic oat plants.
Bio/Technology 10: 1589-1594.
Songstad, D.D., Somers, D.A. and Griesbach, R.J. 1995.
Advances in alternative DNA delivery techniques. Plant
Ceil, Tissue and Organ Culture 40:1 - 15.
Tarczynski, M.C., Jensen, R.G. and Bohnert, H.J. 1993. Stress
protection of transgenic tobacco by production of the osmolyte
mannitol. Science 259:508-510.
Toplet, R., Gronenborn, E., Schafer, S., Schell, J. and
Steinhiss, H.H 1990. Expression of engineered wheat dwarf
virus in seed-derived embryos. Physiology of Plants
79:158-162.
Twell, D., Klein, T.M., Fromm, M.E. and McCormick, S. 1989.
Transient expression of chimeric genes delivered into pollen
by microprojectile bombardment. Plant Physiology
91:1270-1274.
Vaeck, M., Reynaerts, A., Hofte, H., Jensen, S., De
Beuckeller, M., Dean, C., Zabeau, M., Van Montagu, M. and
Leemans, J. 1987. Transgenic plants protected from insect
attack. Nature 328:33-37.
Vasil, I.K. 1995. Cellular and molecular genetic improvement
of cereals. In: Current Issues in Plant Molecular and
Cellular Biology. Terzi, M. et al. (Eds.), pp.
5-18. Kluwer Academic Publishers, Netherlands.
Vasil, V., Castillo, A.M., Fromm, M.E. and Vasil, I.K. 1992.
Herbicide resistant fertile transgenic wheat plants obtained
by microprojectile bombardment of regenerable embryogenic
callus. Bio/Technology 10:667674.
Wan, Y. and Lemaux, P.G. 1994. Generation of large numbers of
independently transfonned fertile barley plants. Plant
Physiology 104:37-48.
Wilmink, A. and Dons, J.J.M. 1993. Selective agents and marker
genes for use in transformation of monocotyledonous plants.
Plant Molecular Biology Report. 11:165185.
Weeks, J.T., Anderson, O.D. and Blechl, A.E. 1993. Rapid
production of multiple independent lines of fertile transgenic
wheat (Triticum aestivum). Plant Physiology
102:1077-1084.
Xu, X. and Li, B. 1994. Fertile transgenic indica rice plants
obtained by electroporation of the seed embryo cells. Plant
Cell Reproduction 13:237-242.
Copyright 1995 African Crop Science Society
|